Silicon carbide ceramic material
With the development of science and technology, especially the rapid development of energy and space technology, materials are often required to have superior properties such as high temperature resistance, corrosion resistance, and wear resistance in order to be used in harsh working environments. Special ceramic materials have become cutting-edge due to their excellent properties such as strong oxidation resistance, good wear resistance, high hardness, good thermal stability, high high-temperature strength, small thermal expansion coefficient, high thermal conductivity, and resistance to thermal shock and chemical corrosion. An important part of science that is universally valued.
Silicon carbide ceramics are a new material that has only begun to develop in the past twenty years. However, due to its particularly excellent high strength, high hardness, corrosion resistance, and high temperature resistance, it has been quickly developed and used in petrochemical and metallurgical industries. Machinery, aerospace, microelectronics, automobiles, steel and other fields, and increasingly show advantages that other special ceramics cannot match.
The rapid development of modern national defense, nuclear energy and space technology, as well as the automobile industry and marine engineering, has placed increasingly high demands on materials such as rocket combustion chamber linings, aircraft turbine engine blades, nuclear reactor structural components, high-speed pneumatic bearings and mechanical seal parts. A variety of new high-performance structural materials need to be developed.
Silicon carbide (SiC) ceramics have excellent properties such as high high-temperature strength, strong oxidation resistance, good wear resistance, good thermal stability, small thermal expansion coefficient, high thermal conductivity, high hardness, and resistance to thermal shock and chemical corrosion. Therefore, it has shown its talents in many fields and is increasingly valued by people.
For example,
SiC ceramics have been widely used in various corrosion-resistant containers and pipes in the petrochemical industry;
It has been successfully used as various bearings, cutting tools and mechanical seal components in the machinery industry;
It is also considered the most promising candidate material in the aerospace and automotive industries for future manufacturing of gas turbines, rocket nozzles and engine components.
Silicon carbide ceramic materials have excellent properties such as high high-temperature strength, strong high-temperature oxidation resistance, good wear resistance, good thermal stability, small thermal expansion coefficient, high thermal conductivity, high hardness, thermal shock resistance and chemical corrosion resistance. It is increasingly widely used in automobiles, mechanical and chemical industry, environmental protection, space technology, information electronics, energy and other fields. It has become an irreplaceable structural ceramic with excellent performance in many industrial fields.
Main application areas of SiC ceramics
(1)Abrasive (2) Refractory materials (3)Deoxidizer (4)Military aspect (5) Electricians and electricians (6) Wear-resistant and high-temperature parts (7) Application of silicon carbide ceramics in the preparation of new energy materials (8) The preferred material for precision ceramic components used in photolithography machines (9) Filtration application of silicon carbide ceramics
7 categories of dry classification equipment and their operating principles
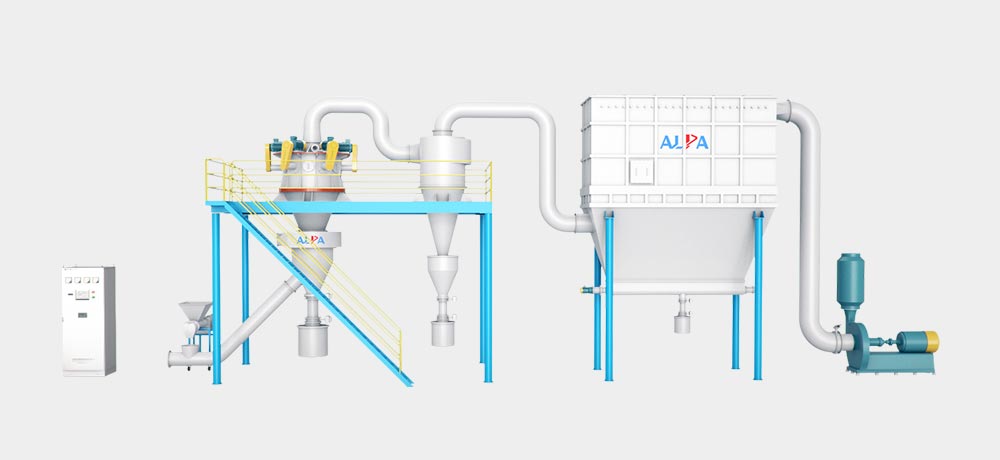
The function of classification is to control the progress of the crushing process and the particle size of the final product. Dry classification is a classification achieved with gas (usually air) as the medium. It is used in water shortage and arid areas, and when the process does not allow the presence of water. , dry grading is the only option. In severe cold areas, the application of dry grading is also not affected. Dry classification saves a lot of water and eliminates the problem of subsequent dehydration in wet classification. It is an effective energy-saving classification method.
Common dry classification equipment includes double impeller air classifier, O-Sepa vortex classifier, cyclone separator, turbine classifier, gravity sedimentation classifier, inertial classifier and jet classifier.
1. Double impeller air classifier
The double-impeller air classifier uses the principles of gravity sedimentation and centrifugal sedimentation to classify, and the product particle size can be as fine as -40 μm.
2. O-Sepa type eddy current classifier
The main structure of the machine includes a spreading plate, impeller, primary air duct, secondary air duct, tertiary air duct, guide blades and shell, etc.
3. Cyclone separator
The cyclone separator is a typical dry centrifugal sedimentation and classification equipment. Its main body consists of an upper cylinder and a lower truncated cone. A core tube is inserted along the central axis from top to bottom at the top of the cylinder, and there is a coarse product outlet at the bottom of the truncated cone. The feed material enters tangentially from the upper part of the cylinder near the outer circumference with the air flow, and is restricted by the shape of the classification chamber to form a swirling motion. The material particles produce radial centrifugal sedimentation motion in the air flow. Coarse particles centrifugally settle at a faster speed, move closer to the cylinder wall, and then slide along the cylinder wall and are discharged from the bottom. The fine particles have a slow centrifugal sedimentation speed, are suspended close to the axis, and then enter the core tube with the air flow and are discharged upward. There are many improved products in practical applications to adapt to different grading requirements and obtain higher grading performance. The classification particle size of the cyclone separator is related to its specification (cylinder diameter). The smaller the specification, the finer the classification particle size.
4. Turbine classifier
Turbine classifier is one of the most widely used dry ultra-fine classification equipment at present. It utilizes the principle of centrifugal sedimentation classification. Its main working component is the turbine (grading wheel), which is equipped with many blades to form a radial gap.
5. Dry gravity sedimentation classification equipment
The main dry gravity sedimentation classification equipment includes horizontal flow type, vertical flow type and meandering flow type gravity classifiers, etc., all of which are used in the ultra-fine stage.
6. Dry inertia classification equipment
The main dry inertial classification equipment includes linear, curved, louver and K-type inertial classifiers, with cut-point particle sizes ranging from 0.5 to 50 μm.
7. Jet classifier
Jet classifier is a dry ultra-fine classification equipment that utilizes jet technology, inertia principle and Coanda effect. Jet technology is used for feeding materials, which allows the feed particles to obtain the necessary inlet velocity and allows the air flow to better produce a Coanda effect. The Coanda effect is when there is surface friction between a fluid (liquid or gas) and the surface of the object it flows through, causing the fluid to slow down. As long as the curvature of the surface of the object is not too large, according to Bernoulli's principle in fluid mechanics, the slowdown in flow speed will cause the fluid to be adsorbed on the surface of the object.
Five common types of modification methods for kaolin clay
In the application process of kaolin, modification is an important deep processing method. It is based on the active groups of kaolin (including aluminum alcohol groups, silanol functional groups, etc.) and changes the process characteristics of kaolin through mechanical, physical, and chemical methods. , to meet its application requirements in production in various fields and industries.
1. Thermal modification
Thermal modification mainly removes part or all -OH from the surface of kaolin through high-temperature calcination, thereby changing the surface properties of kaolin and making it have higher whiteness, better insulation and thermal stability. Applying it as a filler to coatings, rubber, plastics, and paints can improve the performance of the corresponding products.
2. Acid-base modification
Acid modification means that during the calcination process of kaolin, the chemical environment of Al in the phase change process is different, making the Al in it have acid reactivity. Alkali modification means that during the calcination process of kaolin, the chemical environment of Si is different during the phase change process. The SiO2 in the kaolin is calcined at high temperature to activate it, so that the activated silicon in the kaolin reacts with alkaline substances to achieve the purpose of modification.
After acid-base modification, the pore size of kaolin increases, the pore distribution is more concentrated, and the specific surface area is greatly increased. Using acid-base modified kaolin as a filler can improve the air-tightness performance of composite materials.
Surface modification refers to the process of coating some organic or inorganic substances on the surface of kaolin particles through physical or chemical adsorption, thereby modifying kaolin. It is currently the most important modification method of kaolin. Commonly used surface modifiers mainly include silane coupling agents, silicone (oil) or silicone resin, surfactants and organic acids.
Silane coupling agent is the most commonly used and effective surface modifier for kaolin fillers. The treatment process is relatively simple. Generally, kaolin powder and prepared silane coupling agent are added to the modification machine for surface coating treatment. The process can be carried out continuously or in batches.
After surface modification, kaolin has good hydrophobicity and lipophilicity, better dispersion in the polymer matrix, is less likely to agglomerate, and has better compatibility with the polymer. The surface-coated kaolin is used as filler to fill plastics, rubber and other polymers in order to improve the mechanical properties and gas barrier properties of plastics and rubber composites.
4. Intercalation modification
Due to its special structure, kaolin has hydrogen bonds between layers and strong covalent bonds within the layers, and the two sides of the layers are respectively the silicon-oxygen tetrahedron atomic layer and the aluminum-oxygen octahedron hydroxyl layer, so there are only a few highly polar ones. Only substances with small molecular weight can be inserted into the kaolin layers, such as DMSO, formamide (FA), potassium acetate, hydrazine, etc. Other organic macromolecules require two or more intercalations to enter the kaolin layers. What's more, The latter needs to be inserted into the kaolin layer by displacement or entrainment of the precursor.
Intercalation modification technology is a kaolin surface modification technology that is widely used in the preparation of nanoscale kaolin. After intercalation, the distance between kaolin layers increases. After intercalation and peeling, the kaolin particle size is smaller and the specific surface area is larger. Using kaolin that is first intercalated and then peeled off as a filler to improve the air-tightness of composite materials is currently an important method to improve the air-tightness of composite materials.
5. Mechanochemical modification
The mechanochemical modification method essentially uses mechanical energy to activate particles and surface modifiers to achieve the purpose of converting mechanical energy into chemical energy. This can be achieved through strong mechanical stirring, impact, grinding, etc., or with the help of external mechanical force. The surface of the powder particles is coated with a layer of finer or functional powder particles. The mechanical chemical modification method uses different machines and modification processes, so the modification effects of the powder are also different.
The thermal conductivity of thermal interface materials is related to fillers
Thermal interface materials are not only widely used for heat dissipation of electronic equipment, but also have increasing demand in 5G communications, new energy vehicles, etc. In addition, they also have broad application prospects in the fields of military equipment and aerospace.
As a type of thermally conductive material, thermal conductivity is naturally the most important technical indicator of thermal interface materials. Commonly used thermal interface materials are mainly filled types, which are mainly prepared by filling a polymer matrix with high thermal conductivity fillers.
Usually, the inherent thermal conductivity of the polymer matrix is relatively low (about 0.2W/(m·K)). Therefore, the thermal conductivity of the thermal interface material is often determined by the filler.
Different types have different thermal conductivity
Commonly used thermally conductive fillers can be mainly divided into: metal thermally conductive fillers, carbon material thermally conductive fillers, and inorganic thermally conductive fillers.
Metals have good thermal conductivity and high thermal conductivity, so they are a commonly used thermal conductive filler. Commonly used metal thermally conductive fillers mainly include gold powder, silver powder, copper powder, aluminum powder, zinc powder, nickel powder and low melting point alloys.
Carbon materials generally have extremely high thermal conductivity, even better than metal fillers. The inherent thermal conductivity of the added carbon filler is one of the most important parameters that determines the thermal conductivity of carbon-based polymer composites. Commonly used carbon materials include graphite, carbon nanotubes, graphene, expanded graphite, carbon fiber and carbon black. Among them, carbon nanotubes have a thermal conductivity of 3100-3500W/(m·K) and graphene has a thermal conductivity of 2000-5200W/(m·K), making them promising candidates for thermal management applications.
Ceramic fillers not only have good thermal conductivity, but also have relatively low electrical conductivity. They are currently the most widely used fillers. Commonly used ceramic fillers mainly include oxides and nitrides. Oxides include Al2O3, ZnO, MgO, etc.; nitrides include: AlN, BN, etc.
Different shapes, different thermal conductivity
Thermal conductive fillers come in various shapes such as spherical, irregular, fibrous and flaky. Compared with zero-dimensional materials, one-dimensional materials (such as carbon nanotubes, carbon fibers, etc.) and two-dimensional materials (such as graphene, hexagonal boron nitride, flaky alumina, etc.) with ultra-high aspect ratios can be used in The larger contact area formed between fillers provides a wider path for the transmission of phonons, reduces the interface contact thermal resistance, and is conducive to the construction of a thermal conductive network in the system. However, since spherical fillers do not cause a sharp increase in viscosity at high filling levels, they are the most widely used in industry.
Different sizes, different thermal conductivity
The size of the thermally conductive filler also has a significant impact on the thermal conductivity of the thermally conductive composite.
When the filler is of a single size and the filling amount is the same, the thermal conductivity of composites filled with large particle size fillers tends to be higher than that of composites filled with small particle size fillers. This is because there is less interface contact between large particles. The interface thermal resistance is low. However, the particle size cannot be too large, otherwise, the fillers cannot form a close packing, which is not conducive to the formation of thermal conductive paths.
Different degrees of surface modification have different thermal conductivity
In order to solve the problem of interfacial thermal resistance, surface chemical functionalization of fillers is considered to be an effective method. Surface chemical functionalization of fillers can form covalent bridges that improve interfacial adhesion and minimize interfacial phonon scattering by interconnecting particle-resin and particle-particle interfaces. To improve the thermal conductivity of polymer composites, surface treatments have been applied to different fillers such as boron nitride nanotubes, graphene, etc.
Different purity and different thermal conductivity
Impurities in the filler will not only affect the electrical properties of the thermal interface material, but also have a certain impact on the process performance.
Differences in application of sapphire wafers with different crystal orientations
Sapphire is a single crystal of aluminum oxide. It has a trigonal crystal system and a hexagonal structure. Its crystal structure is composed of three oxygen atoms and two aluminum atoms combined by covalent bonds. It is arranged very tightly and has strong binding chains and It has high lattice energy and almost no impurities or defects inside the crystal, so it has excellent electrical insulation, transparency, good thermal conductivity and high rigidity properties, and is widely used as optical windows and high-performance substrate materials. However, the molecular structure of sapphire is complex and anisotropic. The processing and use of different crystal orientations have very different effects on the corresponding physical properties, so the uses are also different. Generally speaking, sapphire substrates are available in C, R, A and M plane orientations.
Application of C-side sapphire
As a third-generation wide-bandgap semiconductor, gallium nitride (GaN) material has properties such as wide direct band gap, strong atomic bonds, high thermal conductivity, good chemical stability (almost not corroded by any acid) and strong With excellent radiation resistance, it has broad prospects in the application of optoelectronics, high-temperature high-power devices and high-frequency microwave devices. However, due to the high melting point of GaN, it is currently difficult to obtain large-sized single crystal materials. Therefore, a common method is to perform heteroepitaxial growth on other substrates, which has higher requirements for substrate materials.
Application of A-side sapphire
Due to its excellent comprehensive properties, especially its excellent transmittance, sapphire single crystal can enhance the penetration effect of infrared rays, making it an ideal mid-infrared window material and has been widely used in military optoelectronic equipment. Among them, the A-side sapphire is the surface in the normal direction of the polar surface (C-side) and is a non-polar surface. Generally, the quality of sapphire crystals grown in the a direction is better than that of crystals grown in the c direction. It has fewer dislocations, less mosaic structures and a more complete crystal structure, etc., so it has better light transmission performance. At the same time, due to the A surface The atomic bonding method of Al-O-Al-O makes the hardness and wear resistance of a-direction sapphire significantly higher than that of c-direction. Therefore, A-direction wafers are mostly used as window materials; in addition, A-direction sapphire It also has uniform dielectric constant and high insulation properties, so it can be used in hybrid microelectronics technology and can also be used for the growth of high superconductors.
Application of R-surface/M-surface sapphire
The R plane is the non-polar plane of sapphire. Therefore, changes in the position of the R plane in sapphire devices give it different mechanical, thermal, electrical and optical properties. Generally speaking, R-plane sapphire substrates are preferred for heteroepitaxial deposition of silicon, mainly for the manufacture of semiconductor, microwave and microelectronic integrated circuit applications. R-type substrate growth can also be used. With the current popularity of smartphones and tablet computer systems, R-surface sapphire substrates have replaced existing compound SAW devices used in smartphones and tablet computers, providing a device substrate that can improve performance.
In addition, when the R-plane or M-plane is used to grow non-polar/semi-polar epitaxial layers, compared with the C-plane sapphire substrate, it can partially or even completely improve the problems caused by the polarization field in the light-emitting device. Therefore, the substrate material used as LED can help to improve the luminous efficiency. However, when processing or cutting, choosing the m-face as the cutting surface is prone to cracking, and it is difficult to prepare a high-quality surface.
How to choose a suitable jet mill based on powder characteristics?
With the development of industrial technology, micro-nano powders have special volume effects and surface effects, and their optical, magnetic, acoustic, electrical and mechanical properties are very different from normal conditions, and are used as the key to many new functional materials. Based on the basic raw materials, the corresponding micro-nano powder processing technology has also achieved unprecedented development. Jet mills (jet mills) use high-speed airflow to cause materials to collide, impact, and shear with impact components. They can not only produce fine particles with narrow distribution, but also have clean and smooth particle surfaces, regular particle shapes, good dispersion, and high activity. of micro-nano powder, and the entire crushing system adopts a closed crushing mode to reduce dust pollution and at the same time, the degree of contamination of the crushed materials is small.
However, since there are many types of airflow pulverizers with different working principles and different crushing effects for various materials, it is necessary to choose a suitable airflow pulverizer according to different materials. At present, according to the different structures or working methods of jet mills, they can usually be divided into: collision type, flat type, fluidized bed type, circulating tube type and target type, etc. On this basis, it can also be classified according to the material characteristics. , adopting methods such as low-temperature cryogenic airflow crushing and inert gas protection to further optimize the crushing effect of the airflow grinder.
Collision airflow crusher
Opposing jet mills are also called opposing jet mills and reverse jet mills. When the equipment is working, two accelerated materials and high-speed airflow meet at a certain point on the horizontal straight line and collide to complete the crushing process. The crushed fine particles enter the external classifier with the airflow under the action of the classification rotor, and pass through the airflow. solid separation and become a product. The coarse particles remain at the edge of the classification chamber and return to the crushing chamber for further crushing until they meet the particle size requirements and enter the external classifier.
Spiral jet pulverizer
Spiral jet mill, also known as horizontal disc jet mill, It is the earliest and most widely used jet mill in industry. The main component of a conventional flat airflow mill is a disc crushing chamber, around which are arranged several (6 to 24) high-pressure working fluid nozzles, Venturi tube feeders, finished product collectors, etc. at a certain angle. The material to be crushed enters the venturi tube driven by the gas. Using the special structure of the venturi tube, the material is accelerated to supersonic speed and then enters the crushing chamber. In the crushing chamber, the materials move in a circular motion driven by high-speed swirling flow. The particles, particles and the inner wall of the machine impact, collide, and rub against each other to be crushed. The coarse particles are thrown towards the peripheral wall of the crushing chamber due to centrifugal force for circulation and crushing, while the fine particles enter the cyclone separator and are collected under the action of centrifugal airflow.
Fluidized bed jet pulverizer
Fluidized bed airflow mill is currently the leading model of airflow pulverizer. It mainly combines the principle of counter-jet with the expanding gas jet flow in the fluidized bed. It is commonly used in the production of chemical raw materials, medicines, cosmetics, advanced ceramics, magnetic powder and other materials. . When the equipment is working, air is sprayed into the crushing area through several reverse nozzles, and the materials to be crushed are accelerated by the high-pressure airflow in the crushing chamber to form a fluidized state. Then the accelerated materials collide and rub with each other at the intersection of each nozzle to be crushed. The crushed fine materials are carried by the upward airflow to the ultra-fine classifier for classification. The fine materials that meet the product requirements are then collected by the cyclone separator, and the coarse materials are After settling back to the crushing area under the action of gravity, crushing continues.
5 types of commonly used surface modifiers for kaolin clay
After surface modification, kaolin powder can be hydrophobic, reduce surface energy, improve its dispersion and compatibility with polymer base materials, so as to improve the comprehensive performance of polymer base composite materials such as plastics and rubber.
At present, the main modification method of kaolin is surface chemical modification. Commonly used surface modifiers mainly include silane coupling agents, organic silicon (oil) or silicone resin, surfactants and organic acids.
1. Commonly used surface modifiers for kaolin clay
(1) Silane coupling agent
Silane coupling agent is the most commonly used and effective surface modifier for kaolin fillers. Since R of the silane coupling agent is an organophilic group, calcined kaolin can be compatible with organic matrices such as rubber and plastics after surface modification. . When modified kaolin is used as a filler in rubber, the R group will react with the rubber macromolecules during the vulcanization process, so that the kaolin molecules are completely dispersed and integrated into the rubber matrix molecules.
The treatment process using silane coupling agent is relatively simple. Generally, kaolin powder and prepared silane coupling agent are added into the modification machine for surface coating treatment. The process can be carried out continuously or in batches.
The factors that affect the final treatment effect are mainly the particle size, specific surface area and surface characteristics (surface functional groups and activity) of kaolin powder, the type, dosage and usage of silane coupling agent, the performance of modification equipment and the time and temperature of surface modification treatment. wait.
(2) Silicone oil
In addition to silane coupling agents, kaolin used as fillers for wires and cables (such as polyvinyl chloride) is often surface modified with 1%-3% silicone oil. The modification process and equipment are similar to those using silane coupling agents.
Calcined kaolin powder treated with silicone oil is used as a filler for wires and cables. It can not only improve the mechanical and physical properties of the cable, but also improve or enhance the electrical insulation and hydrophobic properties of the cable, and the electrical insulation properties in humid and cold environments. Significant improvement.
(3) Unsaturated organic acids
Unsaturated organic acids, such as oxalic acid, sebacic acid, dicarboxylic acid, etc., can also be used to modify the surface of aminated kaolin powder. This modified kaolin can be used as filler for nylon 66, etc.
(4) Cationic surfactant
For example, octadecylamine can also be used for surface modification of kaolin powder. Its polar groups interact with the surface of kaolin particles through chemical adsorption and physical adsorption. The surface hydrophobicity of kaolin modified by organic amines is enhanced.
(5) Inorganic surface modifier
Titanium dioxide, calcium carbonate, calcium sulfate, etc. can also be used for surface modification of calcined kaolin. The modification method is a surface precipitation reaction in an aqueous solution. After the modified product is washed, filtered and dried, calcined kaolin with a titanium dioxide coating on the surface is obtained.
2. Selection principles of kaolin surface modifiers
The type, dosage and method of use of surface modifiers directly affect the effect of surface modification. Different uses require different types and formulas of surface modifiers.
If we only consider it from the perspective of the interaction between surface modifier molecules and the surface of inorganic powder, of course the stronger the interaction between the two, the better. However, in actual operation, the cost and cost of the modified product must also be considered comprehensively. Application purpose and other factors.
For example, when calcined kaolin is modified and used as a filler for cable insulation rubber and plastics, the dielectric properties and volume resistivity of the surface modifier need to be considered;
If modified kaolin is used as a reinforcing filler for rubber, when selecting a modifier, not only the bonding strength between the modifier and kaolin must be considered, but also the bonding strength between the modifier molecules and the rubber macromolecules. , only when both of them are optimized can the surface modifier have the best modification effect.
For specific application purposes, it is sometimes necessary to use two coupling agents for mixed modification. Utilizing their synergistic effect for modification will achieve unexpected good results. However, attention should be paid to the use method and order of addition of the two modifiers. .
Recycling of ceramic waste materials
The production and consumption of ceramics is increasing year by year, followed by tens of millions of tons of ceramic waste. At the same time, the harm caused by ceramic waste has also been widely criticized. With the widespread spread of concepts such as green development and sustainable development, it is particularly important to convert ceramic waste into recyclable resources.
At present, there are two main ways to reuse ceramic waste resources. One is direct processing to recombine various waste ceramic materials into decorations; the other is to recycle them as raw materials to make different products. Specific applications are as follows:
(1) Recycled handicrafts
Using ceramic waste and other waste generated during the production process as the main raw materials, various ceramic decorative arts are prepared through personalized design and recombination. The texture, pattern, color of the ceramic itself and the irregular patterns produced after the ceramic is broken have unique aesthetic value. These ceramic waste products are combined and processed through aesthetic design to produce handicrafts that can not only protect the environment, but also protect the environment. Unique beauty, it is a good green decorative material. This recycling method has relatively low utilization cost, simple production process, and can be designed to meet people's individual needs, so it has extensive promotion value.
(2) As raw materials for processing
building materials
The main components of ceramic solid waste are silicates, so ceramic waste has certain activity. After treatment, its performance can meet the requirements of active mixed materials and can be used as cement mixed materials. In addition, ceramic solid waste can also be added as aggregate into concrete materials. The use of ceramic waste residue can not only save cement and reduce costs, but also reduce the internal temperature of concrete, enhance later strength, and improve corrosion resistance. Ceramic waste has become an indispensable and important component in the production of high-performance concrete.
Recycle heavy metals
Ceramic waste contains a variety of precious metals, especially silver and palladium, which are highly valuable for recycling. At present, the main methods for extracting precious metals from ceramic waste include liquid-liquid extraction, nitric acid dissolution-sodium carbonate reduction, etc. Recycling precious metals from waste materials to produce high-grade renewable resources not only disposes of waste materials, but also generates considerable economic benefits.
Recycled ceramic tiles
Ceramic waste can also be reused in the production of ceramics itself. For example, waste mud and water can be added to the ingredients of ceramic tiles after being recycled and iron removed. The unglazed green body can also be slurried and reused. The glazed green body waste can be mixed with mud and reused without affecting the glaze firing quality. The waste materials fired at high temperatures can be crushed and reused to recreate ceramics. At present, recycled ceramics from ceramic waste are mainly used to produce ceramic bricks, permeable bricks, antique bricks, porous ceramic plates, etc.
Other uses
Ceramic waste can be used to make fireproof and heat-insulating materials, and can also be used to make new ceramic building materials, such as sound-absorbing materials, shock-absorbing materials, water storage materials, etc. Piezoelectric ceramic waste can be mixed into damping and vibration-damping materials such as asphalt and rubber to improve the material's vibration-damping performance.
What are the requirements for alumina powder in high value-added applications?
Alumina high-density particles for sapphire crystal growth
In fact, sapphire is an alumina single crystal. Its growth uses high-purity alumina powder with a purity of >99.995% (commonly called 5N alumina) as a raw material. However, due to the small packing density of micronized alumina particles, it is generally Less than 1g/cm3, the charging amount of a single furnace is small, which affects the production efficiency. Generally, the alumina is densified into high-density particles through appropriate treatment before charging to grow crystals.
Nano-alumina abrasives for CMP polishing abrasives
Currently, the commonly used CMP polishing fluids include silica sol polishing fluid, cerium oxide polishing fluid and alumina polishing fluid. The first two have small abrasive grain hardness and cannot be used for polishing high-hardness materials. Therefore, the oxide polishing fluid with Mohs hardness of 9 Aluminum is widely used in the precision polishing of sapphire fairings and flat windows, crystallized glass substrates, YAG polycrystalline ceramics, optical lenses, high-end chips and other components.
The size, shape, and particle size distribution of abrasive particles all affect the polishing effect. Therefore, alumina particles used as chemical mechanical polishing abrasives should meet the following requirements:
1. In order to achieve angstrom-level flatness, the alumina particle size must be at least 100nm and the distribution must be narrow;
2. In order to ensure hardness, complete α-phase crystallization is required. However, to take into account the above particle size requirements, sintering needs to be completed at a lower temperature to avoid complete α-phase transformation while the grains grow.
3. Since the polishing of wafers has extremely high purity requirements, Na, Ca, and magnetic ions need to be strictly controlled, up to ppm level, while radioactive elements U and Th need to be controlled at ppb level.
4. Polishing fluids containing Al2O3 have low selectivity, poor dispersion stability, and easy agglomeration, which can easily cause serious scratches on the polishing surface. Generally, modifications are required to improve its dispersion in the polishing fluid to obtain good polished surface
Low-alpha emissive spherical alumina for semiconductor packaging
In order to ensure the reliability of semiconductor devices and enhance the core competitiveness of products, it is often required to use Low-α ray spherical alumina as packaging material. On the one hand, it can prevent the operation failure of memory devices caused by α rays, and on the other hand, it can utilize its high heat The conductivity provides good heat dissipation performance for the device.
Alumina transparent ceramic
First of all, in order to prevent impurities in Al2O3 powder from easily forming different phases and increasing the scattering center of light, resulting in a reduction in the intensity of the projected light in the incident direction, thereby reducing the transparency of the product, the purity of Al2O3 powder is required to be no less than 99.9%, and It should be α-Al2O3 with a stable structure. Secondly, in order to weaken its own birefringence effect, its grain size should also be reduced as much as possible. Therefore, the particle size of the powder used to prepare alumina transparent ceramics should also be less than 0.3 μm and have high sintering activity. In addition, in order to avoid agglomeration into large particles and losing the advantages of the original small particles, the powder should also meet the requirements of high dispersion.
High frequency communication alumina ceramic substrate
High-purity alumina ceramics are currently the most ideal and most widely used packaging substrate material due to their good dielectric properties, rigid load-bearing capacity, and resistance to environmental erosion. However, the main performance of alumina substrates increases with the increase in alumina content. In order to meet the needs of high-frequency communications, the purity of alumina ceramic substrates is required to reach 99.5% or even 99.9%.
Sintered NdFeB manufacturing process-jet mill
Jet mill (JM) powder making is a new type of powder making method that uses high-pressure airflow (usually high-purity nitrogen) to accelerate powder particles to supersonic speed in the airflow grinding chamber, causing the powder particles to collide with each other and break.
The specific process is: mix the crushed hydrogen flakes (SC) with a certain proportion of antioxidant, then add it to the airflow mill feeding bin, add it to the airflow grinding chamber according to the quantitative amount, and high-pressure nitrogen (7kg) is sprayed from the four nozzles of the grinding chamber. , accelerate the material to supersonic speed to form a fluidized bed, and the particles collide with each other and break. The diameter of the broken particles is distributed between 1-8 μm.
Depending on the performance and distribution of the materials, the average airflow milling powder size SMD is between 2.5-4μm. The powder produced by airflow milling is uneven and requires three-dimensional mixing. Before mixing, a certain proportion of lubricant and antioxidants are added to the material tank according to the process to control the oxygen content and improve the molding orientation performance.